Accepted 10 February 2021
Available Online 23 February 2021
- DOI
- https://doi.org/10.2991/mathi.k.210212.001
- Keywords
- Nanofabrication
Janus structure
electrospinning
nanofibers - Abstract
Janus and core–shell nanostructures are the most fundamental structural characteristics for comprising all types of multiple-chamber structures. The facile preparation of Janus structure is one of the most difficult challenges in the field of nanofabrication, whose production can pave a broad way for generating a wide variety of hierarchic nanostructures. Based on several most recent reports, this mini review provides some comments on the generation of Janus nanofibers on the following topics: (1) the key points for fabricating Janus nanofibers using side-by-side electrospinning; (2) the strategies for functionalizing the Janus nanofibers; and (3) the possibilities of complex nanostructures started from the two-chamber Janus structures.
- Copyright
- © 2021 The Authors. Published by Atlantis Press B.V.
- Open Access
- This is an open access article distributed under the CC BY-NC 4.0 license (http://creativecommons.org/licenses/by-nc/4.0/).
1. INTRODUCTION
Nanofabrication is a fast developing field for creating all types of functional nanomaterials. During the past three decades, a wide variety of chemical and physical methods have been developed for nanofabrication accompanying with the developments of nanoscience and nanoengineering.
There are three rich ores for our human kind to achieve knowledge from the nature from a standpoint of materials. One is the grand universe such as distant celestial bodies and the origin of the universe. Another is the things happened at the molecular and nanoscale. The third is the extreme conditions for materials conversions such as extremely high temperature and pressure, or hypermagnetism and absolute vacuum. Correspondingly, from the perspective of understanding the world, the transformation of materials is increasingly focused on nanofabrication for getting new products to meet people’s needs of a better life, particularly those created under extreme conditions such as grapheme and carbon nanotubes fabricated under extremely high temperature.
Nanofabrication needs the supports of new knowledge and theories. In the long history of human science and technology, the most basic and classic theories can be attributed to the interaction theories between matter (such as Newton’s law of universal gravitation) and the transformation theories between matter and energy (such as Einstein’s theory of relativity). Similarly, the theoretical background for nanofabrication is still the transformation of materials to products of nanoscale, and the applied energies for realizing the nanofabrication.
The three most important directions for nanofabrication can be concluded as follows: (1) smaller and smaller to pico-technology; (2) more complex structures of nanodevices; and (3) more orderly nanoproducts such as various inorganic nanotube arrays and aligned nanofibers. Among these directions, the facile and robust fabrication of Janus nanostructures (such as Janus nanofibers and Janus nanoparticles) is one of the most difficult challenges [1,2]. Shown in Figure 1, Janus structure, reflecting a spatial side-by-side relationship of two parts, exists at all material scales, from the earth (one side black and one side white), to a leaf (one side for storage and one side for photosynthesis), to lecithin bi-layer structure of cell wall, to DNA helix structures. In this Janus world, Janus products at nanoscale are presently a hot topic for developing novel functional nanomaterials [3,4]. In this article, several issues about Janus nanofibers such as their fabrication using side-by-side electrospinning, their functionalization strategies, and their further evolution are reviewed.
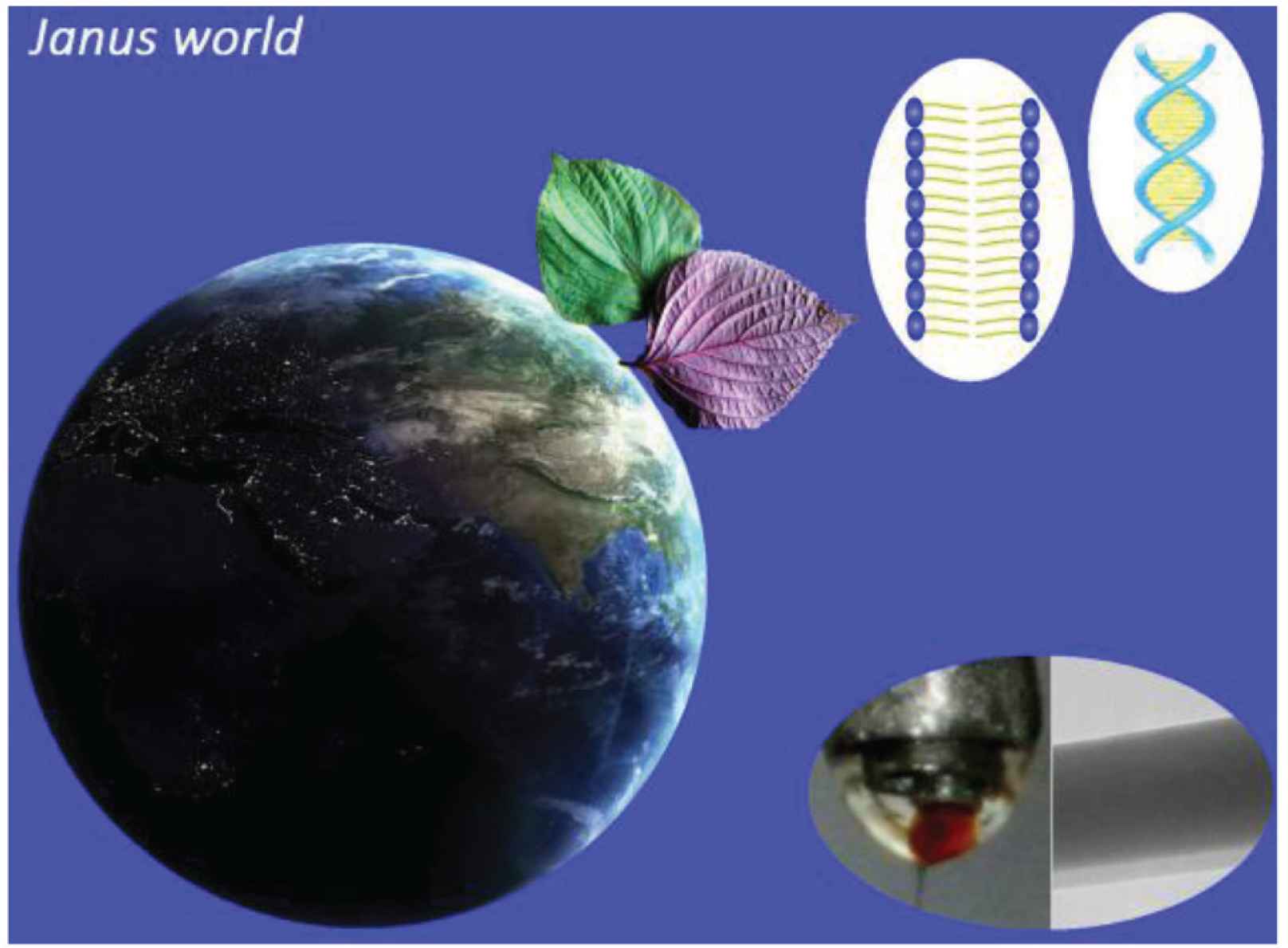
A Janus world from the earth, to a leaf, to the lecithin bilayer structure of cell wall, and to a DNA helix structure.
2. NANOFABRICATION AND KEY POINTS FOR FABRICATING JANUS NANOFIBERS
For nanofabrication, i.e. for creating nanoproducts at nanoscale, there are often two general ways, i.e. top-down way and bottom-up way (Figure 2) [5,6]. Numerous publications have reported the “bottom-up” fabrication methods, by which molecules or building blocks are organized to gather into nanoproducts. One famous method of these bottom-up ways is molecular self-assembly, by which no additional energy is needed for the molecules to aggregate [7–9]. However, these bottom-up nanofabrication ways are often time-consuming and difficult to be scaled-up for possible industrial applications presently.
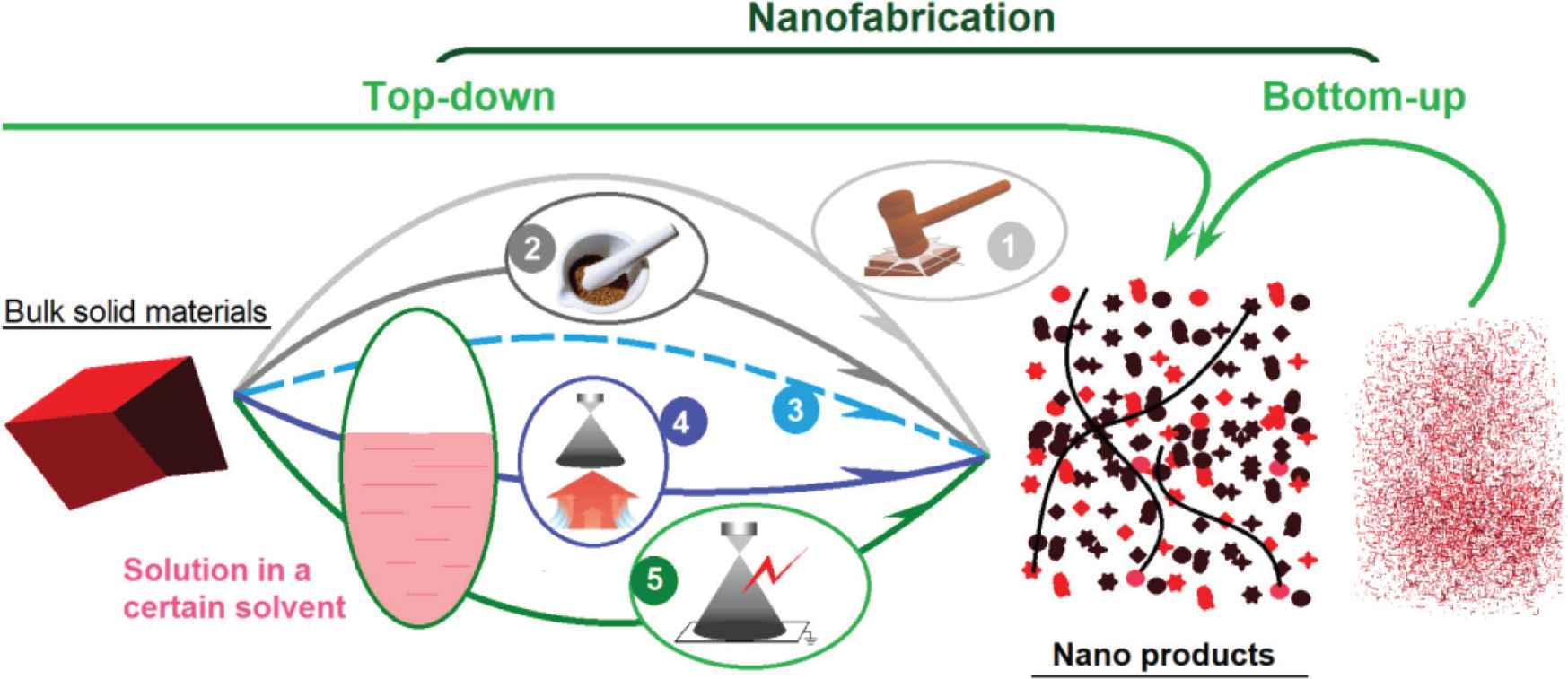
A diagram showing the “top-down” and “bottom-up” nanofabrication routes.
In contrast, the “top-down” ways for nanofabrication holds larger possibilities to produce commercial products. Figure 2 gives a conclusion on these “top-down” ways for nanofabrication. These ways include the traditional (1) hammering, (2) grinding, (3) high pressure homogenization, (4) pressure spraying, and (5) Electrohydrodynamic Atomization (EHDA) methods. The former two methods are conducted by direct mechanical forces on the bulk materials, whose efficiencies are lower and it is very difficult for them to downsize the particles to nanoscale. The energies for the third and fourth methods are still mechanical energies, i.e. high pressure. However, the action mechanisms are totally different. The bulk materials are first transferred into solution, and later the mechanical forces are applied on the solutions, by which the liquid state is easy to be downsized [10,11].
Similarly, EHDA methods take liquid as the treated materials, which include mainly three methods: electrospinning, electrospraying, and e-jet printing [12–15]. But what makes the difference is that the applied energy is high electrostatic energy instead of the mechanical forces. Thus, it is no strange that electrospinning was once called electrostatic spinning. These new methods are presently very popular because of its easiness, efficiency, and utility for nanofabrication. Their products include nanoparticles (electrospraying), nanofibers (electrospinning), beads-on-a-string or spindles-on-a-string hybrids, and 3D-printing articles with fine inner nanostructure properties. Their applications involves a wide range of fields including energy, environmental science and engineering, ceramics, pharmaceutics, cosmetics energy, food industries, and so on [16–18].
All the EHDA processes own the precious capability of drying the working fluids into solid products within several decades of milliseconds, and meanwhile endow the products with a certain outer morphology (for example linear fibers, round particles, concave nanospheres) and a designed inner complicated multiple-chamber structure (such as core–shell and side-by-side). During the past 25 years’ reviving and evolution of electrospinning, it has progressed from the most fundamental single-fluid blending processes to coaxial/side-by-side bi-fluid processes, and to tri-fluid coaxial/side-by-side combined processes [19–22]. Among all the types of electrospinning processes, side-by-side electrospinning for creating Janus nanofibers comprises one of the most difficult challenges, which has also blocked the further developments of multiple-fluid processes.
In several most recent publications, the issue about facile preparation of Janus nanofibers using side-by-side electrospinning has been solved through the usage of a special side-by-side spinneret [23–25], i.e. an eccentric spinneret. The key points for robust and continuous fabrication of Janus nanofibers from the eccentric spinneret can be simple concluded as follows: (1) It has a more reasonable design from a standpoint of “electro”. The two sides are combined into one full circle with one side takes a full moon shape and the other side has a crescent moon shape. Compared with the traditional side-by-side spinneret comprising two parallel metal capillaries, the electrostatic energies can be more efficacious to be transferred into the double working fluids. (2) It provides a larger contact surface area for the two side fluids, and thus ensures an integrated treatment process. In comparison, separation of the two working fluids using a traditional parallel side-by-side spinneret is a common phenomenon due to the small contact surface area and the same charge causing repulsion. (3) The compatibility between the two side fluids is a very important concern for a smooth and robust preparation. The negative phenomena include that (a) the two fluids have a large interfacial tension, by which spinneret clogging would happen frequently; (b) one fluid’s solute would coagulate in the other fluid’s solvent, which would also cause clogging; (c) It is better that the two side fluids have the similar properties such as surface tension and conductivity, which can ensure a matching behavior under the electric field for generating the integrated Janus structures.
3. THE STRATEGIES FOR FUNCTIONALIZING THE JANUS NANOFIBERS
The popularity of electrospun nanofibers has a close relationship with their unique properties such as the small diameter, the huge surface area, the 3D web structure, and the facile tailoring of structure-performance relationship. However, very limited polymeric nanofibers produced by electrospinning are applied as functional nanomaterials. The most common manner for functionalizing nanofibers is to add active ingredient into the filament-forming polymeric matrix. This should be one of the reasons that the single-fluid electrospinning is frequently called blending electrospinning. An active ingredient and a polymer are co-dissolved into a solvent or a solvent mixture to form an electrospinnable working fluid. The working fluid is transferred into a solid nanofiber mat with the active ingredient highly homogeneously distributed all over the polymeric matrix [26–29]. Then, the active ingredient is able to take advantage of the huge surface area of electrospun nanofiber mat for exerting its functional performances such as catalysis, drug release, absorbing, separation, and so on.
Compared with the homogeneous composite nanofibers from a single-fluid electrospinning, the electrospun Janus nanofibers have two different sides to design their functional performances. Meanwhile, compared with the core–sheath nanofibers, Janus nanofibers have their two sides contacting with the environments. Thus, this structure is very useful for designing advanced nanomaterials, by which synergistic effect is needed for an improved functional performance. Interestingly, the recent two publications are similarly about this strategy for developing new sorts of medicated nanofibers.
Wang et al. [24] reports an electrospun zein–Polyvinylpyrrolidone (PVP) nanofiber with both sides loaded with the same active pharmaceutical ingredient, i.e. Ferulic Acid (FA) (Figure 3). When the nanofibers were placed into water, the PVP sides immediately dissolved and freed the loaded FA for an initial therapeutic action. Later, the zein sides, which are insoluble in water, manipulated the sustained release of the residue FA molecules. Thus, the Janus nanofibers are able to provide a two-stage controlled release of FA although it is a poorly water-soluble drug. In the field of pharmaceutics, the dissolution of poorly water-soluble drug is always a big challenge that has drawn increasing attention during the past half century. The electrospun Janus nanofibers not only alter the dissolution behaviors of FA through the polymeric matrices PVP and zein, but also manipulate FA release formats through a combination of PVP and zein in the Janus structures. Based on this strategy, there are numerous possibilities along this way, for example, (1) other kinds of poorly water-soluble drugs can be formulated for furnishing a burst release and a sustained release for the patients’ conveniences; (2) other hydrophilic or soluble polymers can be explored to replace PVP; (3) other insoluble polymers or degradable polymers can be utilized to replace zein for a turnable sustained release time period. Certainly, the drug loading in both sides and the volume of both sides in the Janus structures are also useful parameters to further manipulate the functional performances of electrospun Janus nanofibers. By the way, a Janus fiber-based process–structure–performance relationship is disclosed, which should be useful for developing new functional nanostructures.
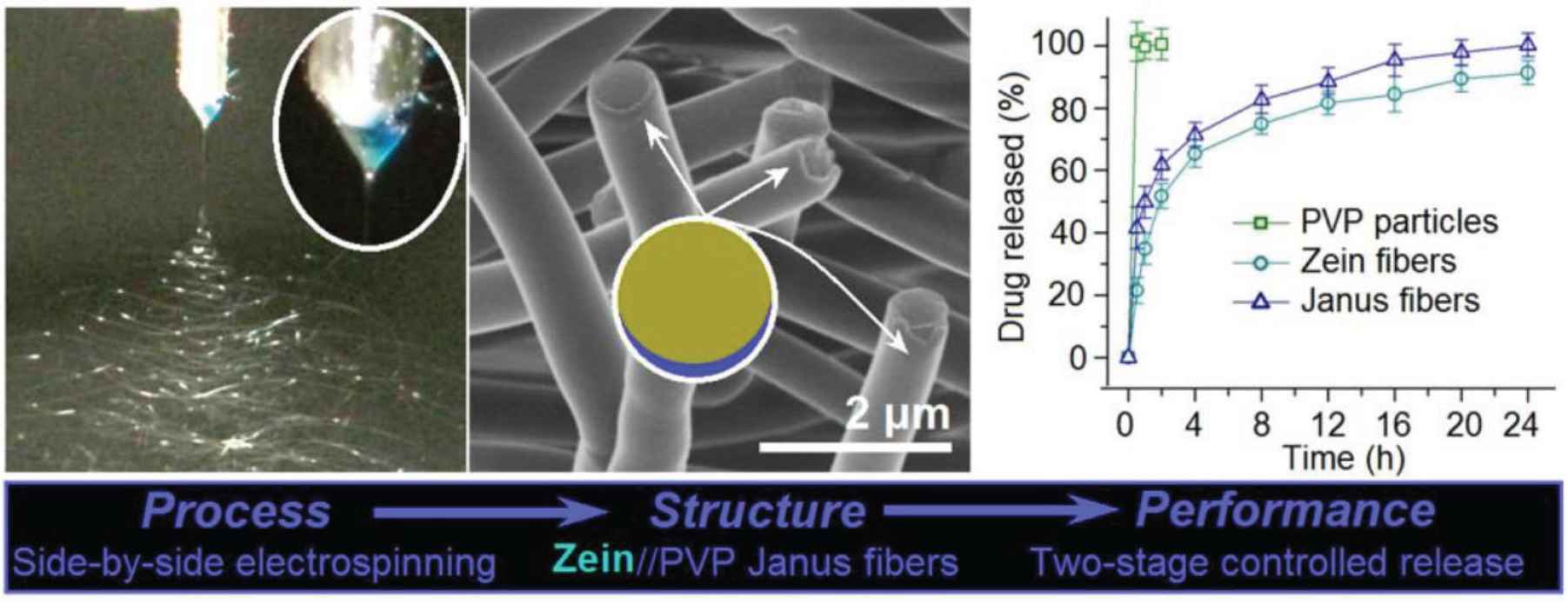
A concept demonstration of Janus nanofibers for providing two-stage drug controlled release profile [24].
Yang et al. [25] recently reports a Janus fiber-based wound dressing. To functionalize the Janus nanofibers, both inorganic active ingredient Silver Nanoparticles (AgNPs) and organic active pharmaceutical ingredient Ciprofloxacin (CIP) are loaded into the Janus fibers (Figure 4). For a fast antibacterial effect, CIP is loaded in the PVP sides. And for a sustained antibacterial effect, AgNPs are loaded in the Ethyl Cellulose (EC) sides. PVP is soluble in water, thus, when the nanofiber mats are placed on the wound places, the CIP molecules would be released all at once to provide the antibacterial action. Later, the AgNPs loaded on the water insoluble EC side would take their roles to give a long-time period antibacterial effect. The authors demonstrated that these PVP–CIP//EC–AgNPs Janus hybrids are able to provide more potent performances in inhibiting the growth of Gram-positive Staphlococcus aureus and Gram-negative Escherichia coli. For wound dressing, the most important performance should be antibacterial effect. This work paves a new strategy for developing high performance wound dressing materials based on the exploitation of side-by-side electrospinning and its related Janus fibers.
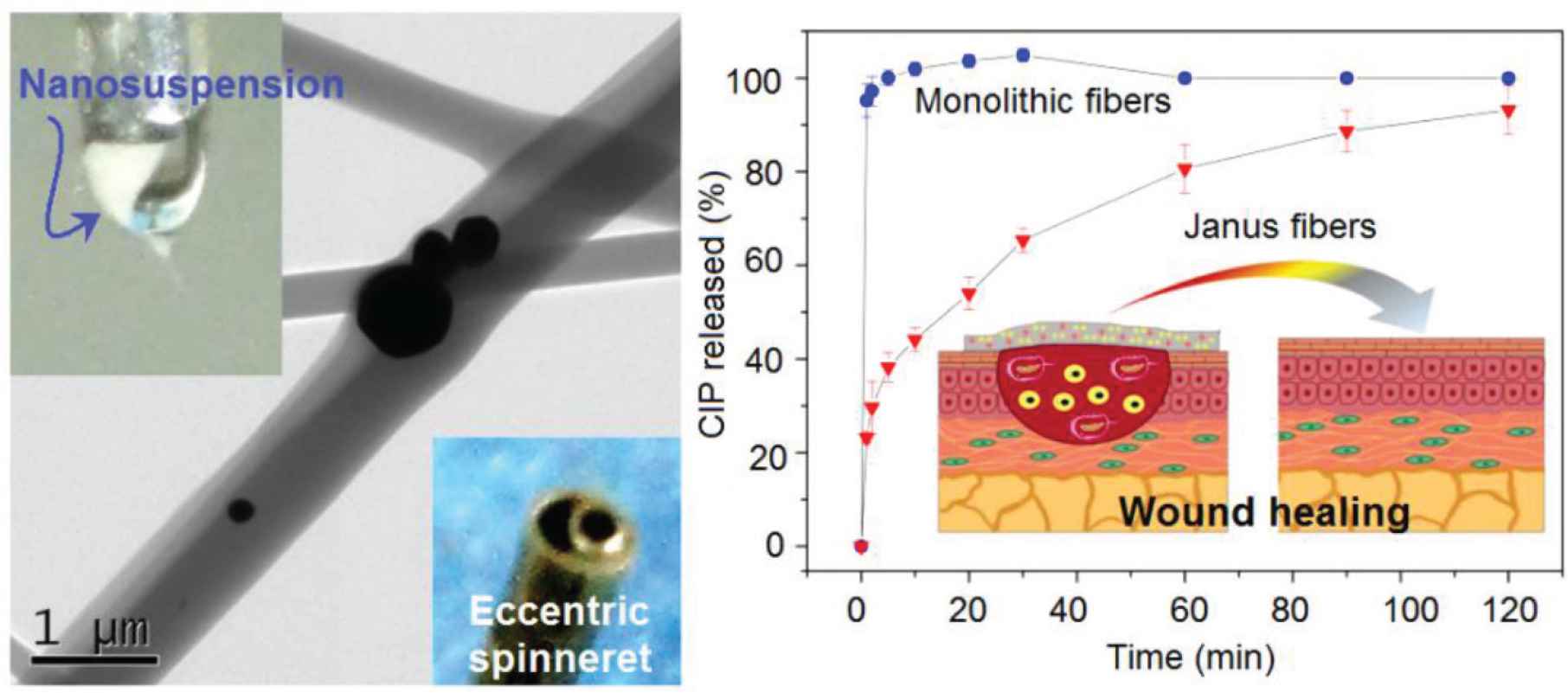
A concept demonstration of electrospun Janus nanofibers loaded with both organic and inorganic active ingredients for a synergistic action on antibacterial performance [25].
4. THE POSSIBILITIES OF JANUS-BASED COMPLEX NANOSTRUCTURES
The development of multiple-fluid electrospinning for creating multiple-chamber nanofibers is very slow, which should has a close relationship with the failure of implementation of side-by-side electrospinning using the traditional two-parallel metal capillaries. Based on the eccentric spinneret, many new kinds of novel spinnerets can be further developed. Correspondingly, the related multiple-fluid processes can be developed because spinneret is the most important section in an electrospinning system.
The preparation of multiple-compartment nanostructures is a big challenge for researchers, which are very useful for developing functional nanomaterials. The popularity of electrospinning is often thought to be its facile and straightforward manner in creating polymeric nanofibers. However, it is another capability of generating complex nanostructure from the macro spinneret makes it a fascinating tool for nanoscience and engineering. Just as core–shell nanostructures can be produced from a concentric spinneret. It is easy to think two parallel metal capillaries spinneret can be explored to create Janus nanofibers. But the electric repulsion often makes the preparation a failure. The most recent reported eccentric spinneret has successfully resolved this problem. Thus, along this way, many new types of multiple-fluid electrospinning processes can be similarly developed. Several examples are shown in Figure 5. The corresponding complex nanostructures prepared from these multiple-fluid processes include Janus structure with one core–shell side (A), tri-layer Janus structure (B), core–shell structure with Janus core (C) [2], and Janus structure with two core–shell sides (D).
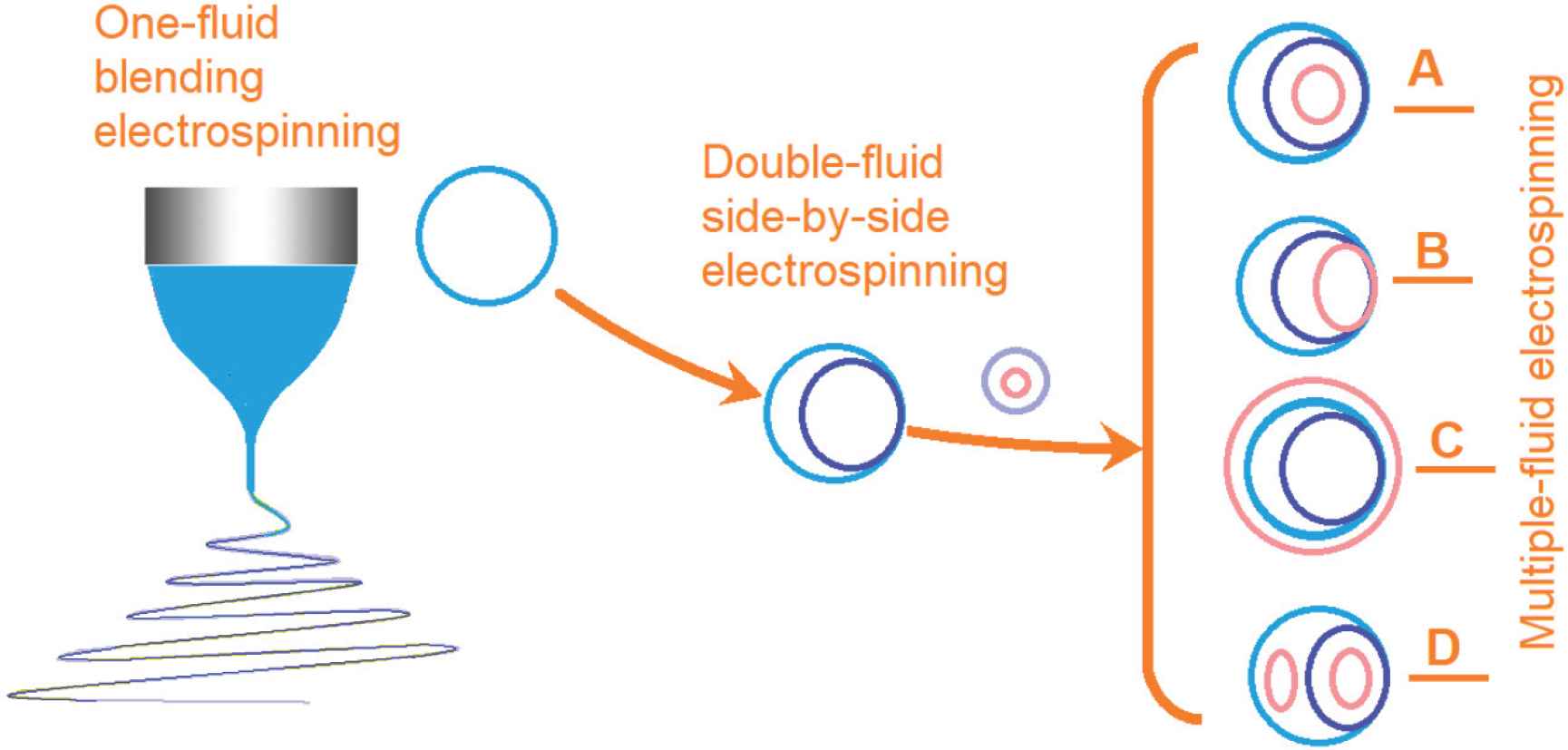
Several possibilities of multiple-fluid electrospinning processes along with the developed double-layer eccentric spinneret: (A) Janus structure with one core–shell side; (B) tri-layer Janus structure; (C) core–shell structure with Janus core; (D) Janus structure with two core–shell sides.
5. CONCLUSION AND PERSPECTIVES
The robust and continuous preparation of Janus nanofibers was once a big challenge in the field of nanofabrication. The most recent reports about eccentric spinneret-based side-by-side electrospinning have shown a simple and useful solution to solve this problem. The limited publications have demonstrated that the electrospun Janus nanostructures are able to provide many strategies to endow and tailor the functional performances of nanofibers. Particularly, the Janus fibers are very useful for designing novel medicated nanomaterials, by which synergistic actions of multiple-components are needed [30].
Based on the eccentric spinneret concept, some new kinds of multiple-fluid electrospinning processes and also side-by-side electrospraying processes [31] can be further developed, and correspondingly some brand-new complex multiple-chamber nanostructures can be created. These complex structures should provide a more powerful platform for developing advanced functional nanomaterials in future [32]. The potential applications or excellent performances that have been demonstrated by two-chamber Janus fibers (such as energy and environment) can be further improved by the new complex structures [33,34]. Meanwhile, as a “top-down” fabrication method, the scalable productions of these complex structures can be realized simply by simultaneous usage of multiple needles, just as those reported large-scale production manners of single-fluid blending electrospinning in literature [35,36].
CONFLICTS OF INTEREST
The authors declare they have no conflicts of interest.
AUTHORS’ CONTRIBUTION
HL and DGY Conceptualization, DGY funding acquisition, DGY project administration, HL, MW and TN writing—original draft, DGY writing—review and editing, All authors have read and agreed to the published version of the manuscript.
ACKNOWLEDGMENT
This work was supported by the Natural Science Foundation of Shanghai (No. 20ZR1439000).
REFERENCES
Cite This Article
TY - JOUR AU - He Lv AU - Deng-Guang Yu AU - Menglong Wang AU - Tingbao Ning PY - 2021 DA - 2021/02/23 TI - Nanofabrication of Janus Fibers through Side-by-Side Electrospinning - A Mini Review JO - Materials Highlights SP - 18 EP - 22 VL - 2 IS - 1-2 SN - 2666-4933 UR - https://doi.org/10.2991/mathi.k.210212.001 DO - https://doi.org/10.2991/mathi.k.210212.001 ID - Lv2021 ER -